Laura L. Calderone/MEDILL Animation: Next Media
Fermi National Accelerator Laboratory is targeting an intense beam of neutrinos from Fermilab in Batavia to Ash River, Minn. This will help them study the properties and behaviors of neutrinos, a particle that could help unlock mysteries of our universe.
BY LAURA L. CALDERONE
MAR 12, 2014
They are one of the universe’s biggest mysteries, yet small to the point of being virtually without mass: neutrinos.
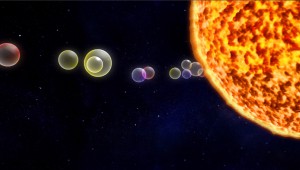
Next Media
Neutrinos are tiny, ghost-like particles that beam down from the sun and other celestial bodies to Earth constantly.
These abundant and miniscule particles could unlock doors to the history of the universe.
“Neutrinos are special because they don’t go away. They are everywhere,” said André de Gouvêa, a particle physics professor at Northwestern University who studies neutrinos.
Hydrogen is the most abundant element in the universe, but for every hydrogen atom there are 10 billion neutrinos, he said. Billions of neutrinos radiate from the sun and other celestial bodies constantly, passing through the Earth – and even you – every day.
You would never know it because these ghost-like particles rarely interact with anything.
“They’re hard to detect, so they’re incredibly hard to study” de Gouvêa said.
Because neutrinos don’t go away – or “decay” – it is possible that some of the neutrinos in the universe may have been present at the time matter was created, he
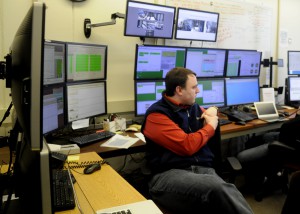
Laura L. Calderone/MEDILL
Physicists at Fermi National Accelerator Laboratory outside of Chicago analyze data coming from subatomic particle the detectors. One pair of new detectors sense when neutrinos interact with scintillator fluid. Neutrinos are very hard to detect because they interact so rarely.
said. This means that the universe could have some neutrinos that were produced during the Big Bang.
The physicists at Fermi National Accelerator Laboratory near Chicago are making strides in the study of these strange particles.
They have begun an experiment, NOvA, which looks for properties of neutrinos to learn more about them and understand the universe better.
Meetings to plan this experiment began nearly 10 years ago, but in February, scientists began seeing their first signs of neutrinos, according to a press release.
“We are proud to reach this important milestone on our way to learning more about these fundamental particles,” said Fermilab Director Nigel Lockyer in a press release.
FermiLab, in conjunction with many other institutions, such as the University of Minnesota, constructed two large detectors to identify the neutrinos as part of the NOvA experiment. One is located at Fermilab in Batavia – the near detector. The other is located 500 miles away in Ash River, Minn., and is called the far detector.
“For scientific reasons we wanted [the far detector] as far from Fermilab as possible. It’s the last road in the United States. If you go past the road at the end of the beam, you will very quickly leave the United States,” said Marvin Marshak, a professor of physics at University of Minnesota and director of the Ash River Laboratory.
Fermilab will send a beam of neutrinos from the near detector to the far detector to study their behavior in great detail, said Peter Shanahan, a scientist at Fermilab.
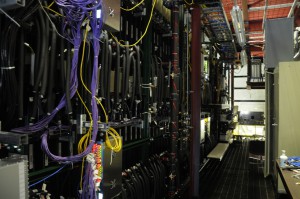
Laura L. Calderone/MEDILL
Detectors sense when neutrinos interact by giving off small amounts of light. The neutrino does not give off light because it is charge neutral. When it interacts it produces other particles, such as electrons, that give off light, according to Peter Shanahan, a scientist at Fermilab.
The beam, called Neutrinos at the Main Injector, or NuMI, will fire the neutrinos straight through the Earth. Because they interact so infrequently with matter, a tunnel for the beam is unnecessary. Some neutrinos are detected in the near detector, but more will likely be detected in the far detector.
The beam’s entire journey will be almost instantaneous, taking less than three milliseconds, according to the Fermilab website.
“Neutrinos, for all intents and purposes, are travelling at the speed of light, technically a little slower because they have mass,” Shanahan said.
The far detector is filled with what scientists call “liquid scintillator,” which is essentially a mineral oil with another chemical in it, according to Shanahan.
“When a charged particle goes through, it gives off a teeny amount of light – like hundreds of photons or less,” he said. “We collect that light on an optical fiber and it gets piped out to a little photo sensor.”
The neutrino is charge neutral, so it won’t give off light, but its interaction with other particles, such as electrons, will give off light. This is how scientists will be able to tell if a neutrino has reached the far detector.
This is an arduous process because neutrinos are reluctant to interact with anything.
“Most, almost all, of the neutrinos that make it into our detectors don’t interact – very, very few,” said Rick Tesarek, a scientist at Fermilab. “Most that go through the Earth, go through our detectors, we never see them.”
The detector’s size and the intensity of the beam increase the odds for neutrino detection. The near detector in Batavia weighs almost 300 tons. The far detector is nearly 47 times the weight of the near detector, weighing in at approximately 14,000 tons, according to Fermilab scientist.
“Think of a five story building, by five story building, by about two thirds of a football field,” Tesarek said.
After the neutrinos are detected, Marshak and the team in Minnesota sends data to Fermilab and all the scientists begin analyzing. While sharing the data takes seconds, the analysis may take years.
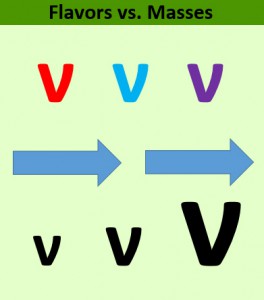
Laura L. Calderone/MEDILL
Neutrinos are known to oscillate from one type, or flavor, to another. Scientists know that there are three types of flavors: muon neutrinos, tau neutrinos and electron neutrinos. They also know that there are three types of masses associated with neutrinos. What makes neutrinos a challenge to study is that scientists don’t know the mass hierarchy. Testing the oscillation of muon neutrinos to electron neutrinos could shed light on this mystery.
One of the things scientists are trying to understand is neutrino oscillation, meaning when one type of neutrino changes to another type of neutrino.
There are three different types, or “flavors,” of neutrinos: muon neutrinos, tau neutrinos and electron neutrinos, based on whether they decay from muons, taus or electrons. An electron is a negatively charged particle. Muons and taus come from the same subatomic particle family as electrons but are more unstable.
“A muon really looks a lot like an electron, except it decays into an electron in just a few microseconds,” said Shanahan. “The tau is really the same thing. It’s heavier. The muon is 200 times heavier than an electron, the tau is even heavier still.”
When muons, taus and electrons decay, one of the things they produce are these tiny neutrinos. Neutrinos comes from an Italian diminutive meaning “little neutral one,” Shanahan said.
Not only do neutrinos have flavors, but they each have a bit of mass, which can be referred to as masses one, two and three. Neutrinos may be virtually massless, but all have slightly different masses.
Where it gets tricky for scientists is that the mass hierarchy from heaviest to lightest neutrino is unknown and very hard to quantify. Increasingly challenging still is that the flavors are not reflective of the masses, de Gouvêa said, who is not affiliated with the current NOvA experiment.
Scientists already know that neutrinos oscillate. By observing the oscillation between neutrinos, scientists can hope to understand the masses.
“We only learn about these masses with neutrino oscillation experiments,” he said. “That’s the only way we have of doing that, in spite of the fact that we’ve tried it other ways.”
Physicist theorize that the origins of these masses could be tied to the big bang. Neutrinos can clue scientists into information about the Big Bang and also help scientists understand the creation of matter and antimatter.
Matter is what we are, what we see and what we touch every day. There is significantly more matter in the universe than its counterpart, scientists said.
“However the universe came into existence, there were equal amounts at least in some instance at the beginning of matter and antimatter,” Shanahan said. “We live in a world, luckily, where there is no antimatter, except for fleetingly in cosmic rays. It’s a big mystery how we got to that.”
Laura L. Calderone/MEDILL
The NOvA experiment plans to study neutrinos changing, or oscillating, from one type to another.
Studying neutrinos and antineutrinos can help scientists learn why there is more matter than antimatter in the universe.
But getting there allowed the universe as we know it to come into being.
Scientists at NOvA in Fermilab started producing muon neutrinos and are looking for them to change into electron neutrinos at the far detector. They plan to eventually switch from producing muon neutrinos to producing muon antineutrinos, an antimatter form of neutrinos. Then scientists will look for electron antineutrinos the far detector, according to Tesarek.
“If those occur at different rates, that would be the signature of a process that would produce this asymmetry between matter and antimatter,” he said.
Understanding these great mysteries is no easy feat.
Neutrino detection has begun, but the far detector’s construction is still incomplete. Scientists say they hope to start publishing research within the next year, but the timeline for the whole NOvA project is over the span of years.